NJIT Chemist Hunts for COVID-19 with a Smartphone-Linked Biosensor
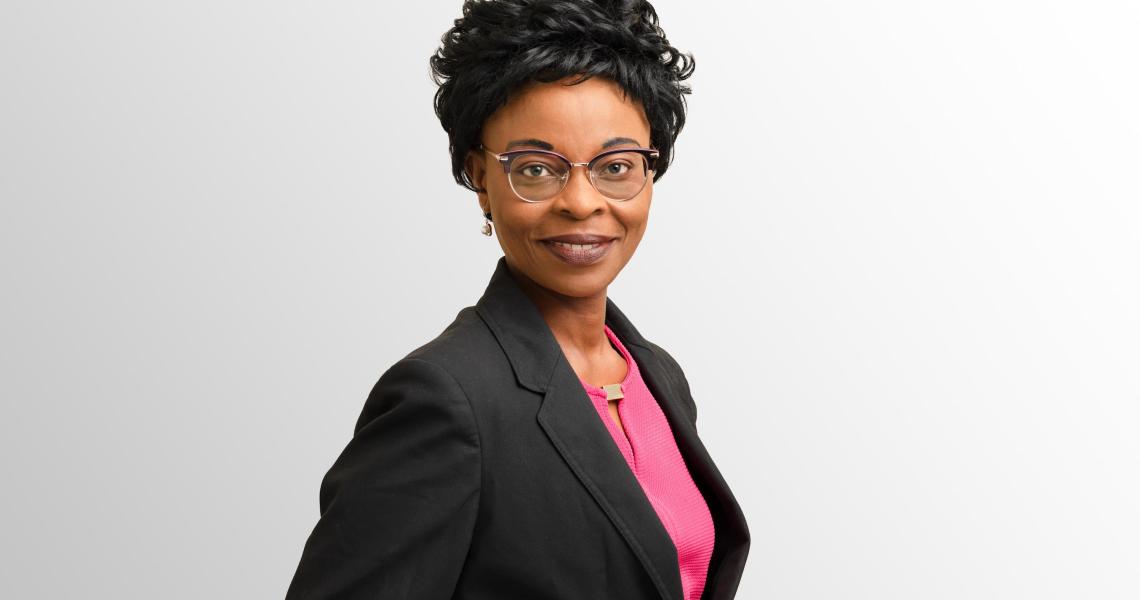
A Q&A with chemist Omowunmi “Wunmi” Sadik
Q: How do chemical sensors work?
Chemical sensors are small devices coated in spots with specific compounds that react to, or recognize, targeted chemicals in the air, water and biological systems. The devices’ transducers convert the results to a measurable signal. They can provide a one-time yes or no answer or deliver continuous information as they monitor changes in the environment or the body. I got my start at the Environmental Protection Agency developing environmental immunosensors that employ antibodies that bind with PCBs — toxic industrial compounds once widely used as insulators — at polluted sites. Sensors are portable and conduct analysis on site, thus eliminating travel and lab-processing time.
Q: How does your newest sensor detect COVID-19?
Most current testing methods for COVID-19 are time-consuming, expensive, vulnerable to false positives and negatives, and often require specialized training. This makes rapid detection difficult. I’m developing a disposable, paper-based biosensor with a modified version of the sugar compound that the coronavirus spike binds with to gain entry into cells. It’s designed as a point-of-care test for saliva that not only detects the presence of the virus, but how much is there, thus giving us a sense of the disease’s progression. It will be read using a smartphone powered by a small rechargeable battery. This device is an adaptation of an earlier sensor I developed and patented to detect E. coli in fresh food in grocery stores and distribution centers.
Q: How does your biosensor improve upon current rapid-testing technology?
The most widely used antigen assays, or rapid tests, use antibodies to bind with — and thus detect — proteins on the surface of the coronavirus that prompt an immune response in the body. They are accurate if the viral load is high, but less so if it’s low, leading to false negative results. What’s different — and unique — about my approach is the sugar-based molecule I designed to bind with a carbohydrate chain attached to a protein on the tip of the coronavirus spike. I tweaked the biochemistry of this chemical compound, which plays a role in the body’s immune system, to increase its affinity for the invading spike protein. The virus bonds more readily with this sugar compound than it does with antibodies, thus enhancing the sensitivity of my test.
Q: How does the device process and transmit test results?
Q: We immobilize my modified molecules onto paper strips and expose them to the test subject’s saliva. The spots on the paper turn various colors depending on the viral load. A network of intertwined cellulose fibers embedded in the paper promotes excellent mechanical, electrical and fluidic properties that allow us to capture and incubate the virus, while an electrochemical biosensor array enables signaling and a simple smartphone readout. The biosensor harvests power from the smartphone and transfers signals through the audio jack, which can then be transmitted to a database at the local, regional or national levels. Ultimately, this tool can be integrated into disease surveillance and response systems for other viruses and emerging pathogens. My device is so simple it can be 3D printed by undergraduates.
Q: What are the challenges in sensor research?
A major hurdle we face is speed. The rate at which we make measurements, then transmit and process that information is very slow. When we take sensors into the field — produce shelves at the grocery store, patients’ rooms at the hospital or factories processing toxic materials — we need data transfer, processing and mining to happen quickly at central locations with large computing capacity. We also need to make sensors smaller, so they can be widely dispersed and are almost invisible. COVID-19, or similar pathogens, could be weaponized, and so these devices would be critical for defense applications, such as potential terror threats in buildings or airports.
Q: What new COVID-related applications are on the horizon?
In the near-term, we will see new technologies that allow us to discover COVID-19 without even touching a person. Our goal is to do detection at the population or community level as an alternative to individual testing. Installing sensors on-site in wastewater systems that transmit that information rapidly will be a first step.
Q: How do your biosensors measure pain?
Pain triggers both cognitive analysis and emotions, making its objective measurement challenging. Determining its intensity, and the underlying cause it signals, is critical not only to effective pain management, but to diagnosis and treatment as well. People addicted to opioids may exaggerate their pain. Children, the unconscious and people with disabilities may not be able to describe their symptoms at all. This is a real problem, because pain is protective — it tells us we’re in trouble. To this end, I’ve developed a biosensor to measure two pain biomarkers, cyclooxygenase-2 (COX-2) and inducible nitric oxide synthase (iNOS), biochemical compounds that appear in the bloodstream when pain is present, including in cancer patients, and have proved sensitive indicators of true pain.
Omowunmi Sadik is distinguished professor of chemistry, chair of the Department of Chemistry and Environmental Science, and director of the BioSensor Materials for Advanced Research and Technology (BioSMART) Center. Her research areas include surface chemistry — interactions at the interface between different molecules; sensors for biological systems and the environment; and new measurement approaches to solve problems such as early diagnosis of cancer, DNA testing, detection of pain biomarkers and food safety.