Designing Dynamic Building Facades For Exchange, Energy and Flow
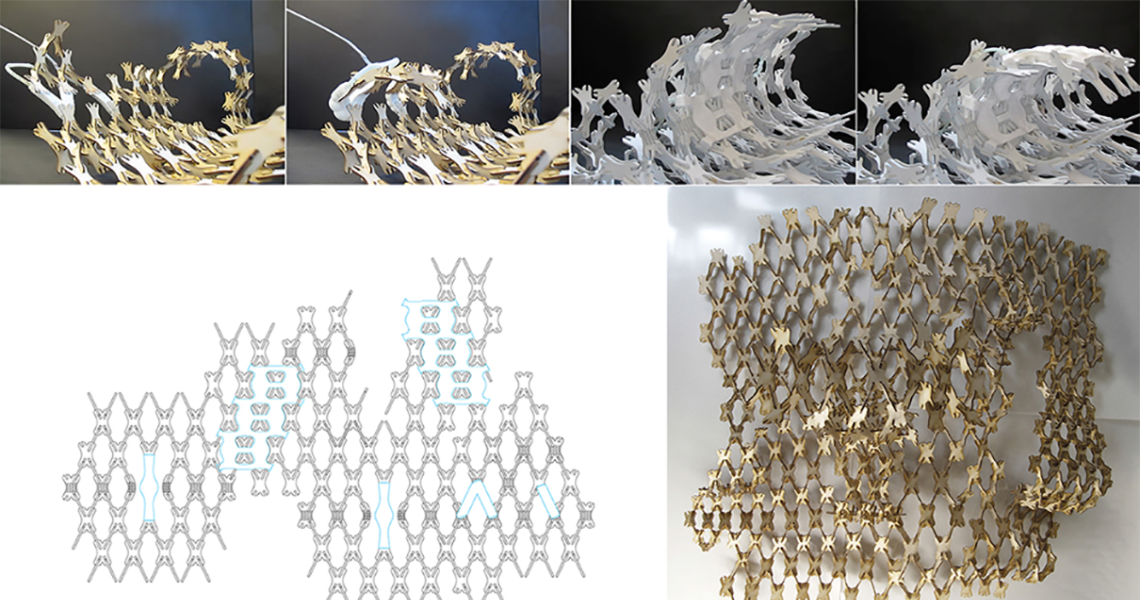
Vera Parlac, associate professor of architecture in the Hillier College, presented her futuristic work on integration of pneumatic muscles into building skins recently at the Façade Tectonics World Congress.
This leading-edge work in the field of building performance focuses on dynamic responsive systems that can adapt to the constantly changing environmental conditions with which they interact. The focus of this research is to attain better performance in building skins adaptative capabilities by producing material systems that have some ‘built-in behaviors’ and are responsive in a way that is similar to living systems.
The applications of these kinds of systems improve performance by reducing the environmental impact caused by the heating and cooling of the built environment, and by improving the ability of the built environment to actively participate in, for example, energy harvesting and to contribute more productively to the broader environment.
The interest in architecture with an adaptive capacity is also causing the definition of resilience in the built environment to adapt as well. What is generally expected from a resilient built environment is a quick return to its original state, prior to degradation or failure influenced by external environmental conditions. Now resilience is being thought of as the ability to adapt to changing conditions, whether immediate or ongoing, in a way that might actually mean an end to the system as it once was.
“To be resilient, the built environment should be designed to adapt to the process of alteration, transformation and change. To go a step further we could think of it as if that process itself should be ‘designed’ and channeled,” said Parlac. “Thinking about resilience in this way can, for example, help us rethink the way we design building skins. When thinking of adaptive building envelopes we could design them to actively harvest sun or wind energy, collect water or shield from excessive heat by dynamically adjusting its elements to actively seek preferred positions.”
This understanding of systems adaptation is well recognized in the natural sciences but in the standards of engineering and architecture practice, as Parlac points out, “Engineered systems or devices that perform specific tasks under predictable external conditions are concerned with constancy of performance. An equilibrium centered view of such systems is static and doesn’t support the transient behavior of natural systems.”
Dormant and active pneumatic muscles
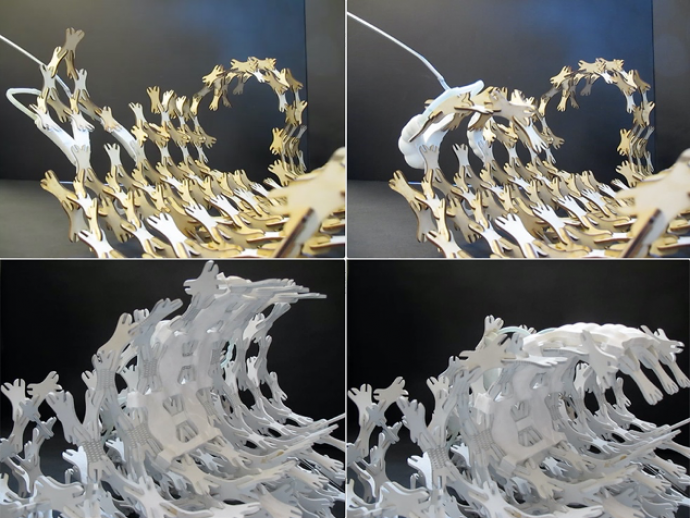
The ability to regulate behavior and adapt to the demands of a situation has always been associated with living organisms. In the design process for dynamic responsive systems in architecture, this focus on a more dynamic and even permeable boundary between engineered systems and natural systems in the environment is taken into account in order to mitigate the harmful effects generated during the construction and life cycle of the built environment.
This adaptive approach requires new kinds of materials and building systems and new ways of thinking about built systems and their dynamic interaction with the environment. This is the focus of Parlacs’ research and pedagogy.
Discussing the project presented at the congress, Parlac said, “I am exploring the integration of custom-made soft robotic muscles with a component-based surface. This project is part of a broader research that focuses on new material behaviors and their capacity to produce adaptive and dynamic material systems by integrating pneu structures to generate kinetic movement within a component-based assembly and produce a responsive programmable architectural skin.”
The aim of this project was to produce a dynamic system that is self-supporting, pliable and kinetic with behavior governed by the configuration of modular components and the position and concentration of pneumatic elements.
“This is a prototype-based exploration that addresses several aspects of a dynamic system. It is a light modular structure with the specific components and a pattern of their aggregation that demonstrates a range of motions achieved by different muscle types. When integrated the light modular structure and inflatable muscles work in unison to produce a dynamic architectural skin.”
Parlac explained the way the system works, “Soft inflatable pneumatic muscles are linked by silicone tubes that allow passage of air through a number of muscles, inflating and deflating them in a sequence. When inflated or deflated, these clusters move entire regions of the modular structure, producing apertures that open, close or shift. The movement of pneumatic muscles depends on the flexibility of the material and the volume of internal chambers and their geometry.”
Through prototyping and iterative design the behavior of the pneumatic muscles as they were integrated into a modular structure was developed and observed. The muscles are designed to be integrated into the structure interchangeably as modular elements.
Building skin study model

A key challenge in the design of dynamic facades is the actuation system that facilitates movement. These systems are often motor based. The main motivation for the development of this system of soft actuation is to produce a building skin that would reduce the presence of complex mechanical systems in dynamic architectural assemblies, such as dynamic building facades.
Parlac adds, “The primary advantage of this mode of actuation is its lightness, as well as the possible degree of movement control that it offers. Systems like these can be used as dynamic shading systems but also as energy harvesting elements capable of responding to the dynamic influences of the sun or wind on a building skin.”
Parlac wants students to understand how the materials and construction industry is complicit in contributing to global warming and how they can develop areas of expertise that will contribute to the transformation of the built environment and a reduction of harm to the natural environment. “On one hand, this will require thinking beyond the concept of sustainability, and becoming familiar with technologies such as distributed computation, embedded computing, sensing technologies, biosensors, material innovation and synthetic biology (all coupled with digital design). On the other hand, a better understanding of how materials are ‘built’ in nature, how resources are used in the natural world and how we affect our environment though our actions are equally important. All of this contributes to the change in thinking and enables integrated strategies that facilitate further development of adaptation and resilience in the built environment.”