Alumnus Robert Cohen Offers an Insider's View of the High-Tech World of Joint Replacement Surgery
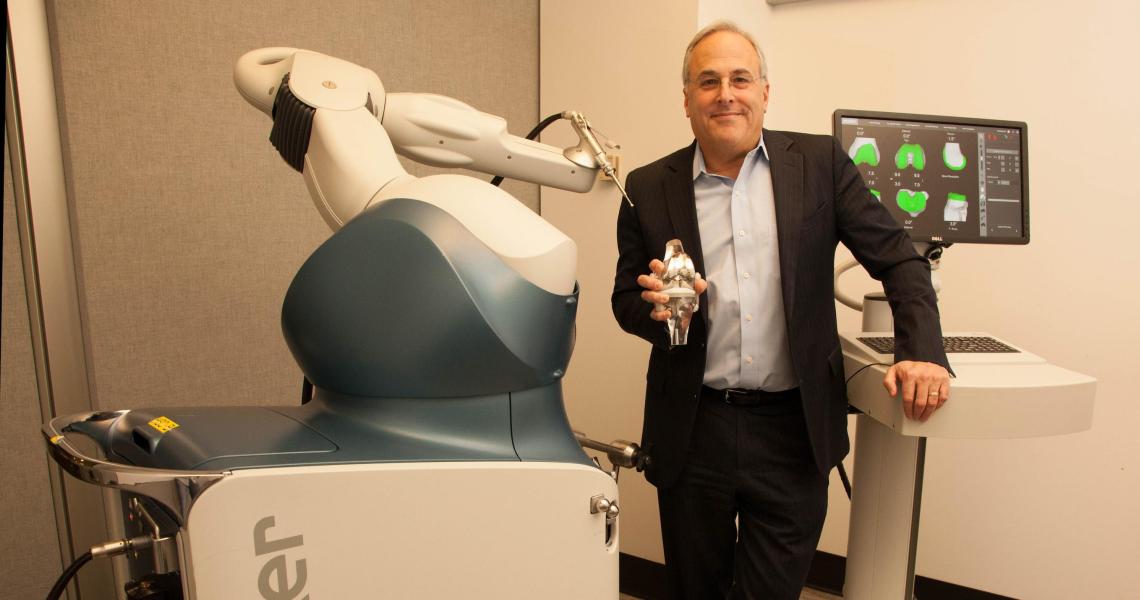
Robert Cohen ’83, ’84, ’87, a biomedical engineer and entrepreneur who specializes in orthopedic joint replacement implants, years ago envisioned the convergence of advanced materials, new fabrication methods and robotic-assisted surgery to maximize motion restoration. In 2010, his New Jersey company, Pipeline Orthopedics, the developer of implants with porous metals designed to improve the fixation of device and bone, entered a strategic alliance with Mako Surgical Corp. in order to use Mako’s robotic systems in the operating room to help place its implants more precisely. During that same era, Pipeline 3D metal-printed one of the first orthopedic devices approved by the U.S. Food and Drug Administration for human orthopedic implantation.
Cohen, a new member of the NJIT Board of Trustees, now heads the 600-person research and development team for the joint replacement division of Stryker, the medical technology giant. Last year alone, 42,000 people underwent robotic-assisted surgeries to implant the company’s knee and hip devices. Below, Cohen discusses the recent burst of innovation in the implant sector, propelled on many fronts by advances in engineering.
Q: What is driving innovation in the joint replacement sector?
A: Expectations are changing. People are living longer, employed longer and physically active longer. In the 1980s – the early days of implant technology – the idea was to permit basic functioning, such as getting out of bed or into a car. Today, people are returning not only to work in many cases, but to tennis and golf. With the relief of joint pain, they want back everything they had in life. Increasingly, they are considering interventions earlier in life, so they don’t have larger problems later.
Q: What are the engineering challenges?
A: People seeking implants want more durable clinical results and a high level of satisfaction in the near- and long-term. For engineers, the challenge is complex. We need to design implants that last a long time, while also striving to give people back the same range of motion they once had. And patients want to feel better as soon as reasonably possible. This means both the implant design and the surgical technique needed to evolve in order to shorten hospital stays and potentially quicken recoveries. As engineers, we will meet these rising expectations with innovations in biomaterials that interact more physiologically in the joint and implant designs that are placed on the bone more precisely to meet individual patients’ needs and function.
Q: What are the key technological advances in biomaterials and device design?
A: We have developed an implant with a porous metal exterior surface made by additive manufacturing. It is produced with a specific 3D printing process known as “direct metal laser sintering” in which a laser is programmed to consolidate titanium alloy powder in a configuration that engineers create with a computer model. The resulting structure is fully solid internally with an external surface porous matrix for new bone to grow into. There are two other important technology advances. We have a growing database of 3D computer models of joint bone images, and, based on this, we can design implants with more information than ever before. These images also guide us in determining where an implant can better fit a person’s anatomy, while restoring that joint’s motion. The 3D metal printing process is such a departure from metal machining methods of the past, that it is allowing us to think differently. We can now shape metal in a way conventional manufacturing never could. We no longer have to think of an implant configuration that only a lathe, a mill or a casting can make. Patients are benefiting from these new 3D-printed implant geometries.
Q: Will you describe a surgery?
A: Before we begin surgery, we now have a very specific idea of exactly where the implant will go in each patient. CT scans of the patient’s bones are converted to a three dimensional computer model. Pre-operatively, the implant models are superimposed over the bone models where the surgeon deems best. That combination implant-bone model is now the preliminary plan and it’s transferred the day of surgery to the Mako system in the operating room. Mako is comprised of a computer and a robotic arm. The cutting instrument that prepares the bone to accept the implant is attached to the end of the arm. The surgeon moves the instrument with the robotic arm, defining the limits of the bone cut surfaces, essentially creating ‘virtual boundaries’ determined by the patient’s hip or knee CT scan and the surgeon’s assessment of the patient intraoperatively. Robotic-assisted surgery provides for implant placement precision and accuracy we have never seen before with other surgical procedure technologies.
Q: What’s on the horizon for implant surgery?
A: We are making advances on several other fronts, including
an effort to make incisions and devices smaller as we design and place implants on individuals’ bones less invasively. The goal is to disrupt less soft tissue and remove less bone. These improvements come at the nexus of evolving robotics, smarter engineering and more sophisticated 3D printing. Looking at the implant sector from the perspective of both an engineer and an R&D executive in a medical device company, we are able to design better implants in unique shapes in conjunction with precise bone preparation that is robotically enabled.
Q: How is the industry-academia nexus in this sector evolving?
A: The partnership between academia and industry has such potential, but progress here is slow. Taking the initiative, we’ve started canvassing universities looking for areas of expertise that can accelerate our development cycle and bring novel medical devices to the market. I think these relationships will prove very useful. Professors are very good at specialization, while the type of labs they run are too speculative and often too expensive for private industry to consider at that early a stage. We are starting to form partnerships with academic labs to work on everything from cell biology, to radiographic image modeling, to
new biomaterials, to 3D printing, to end effectors on robots. Indeed, some of the students we’ve gotten to
know at my alma mater through industry-mentored capstone projects or internships are now working at Stryker. It’s an interesting time in orthopedic joint replacement with new technologies allowing engineers to take a fresh look at everything we do and how we design. Innovation is thriving.