Developing Infrastructure to Last More Than a Century in a Changing World
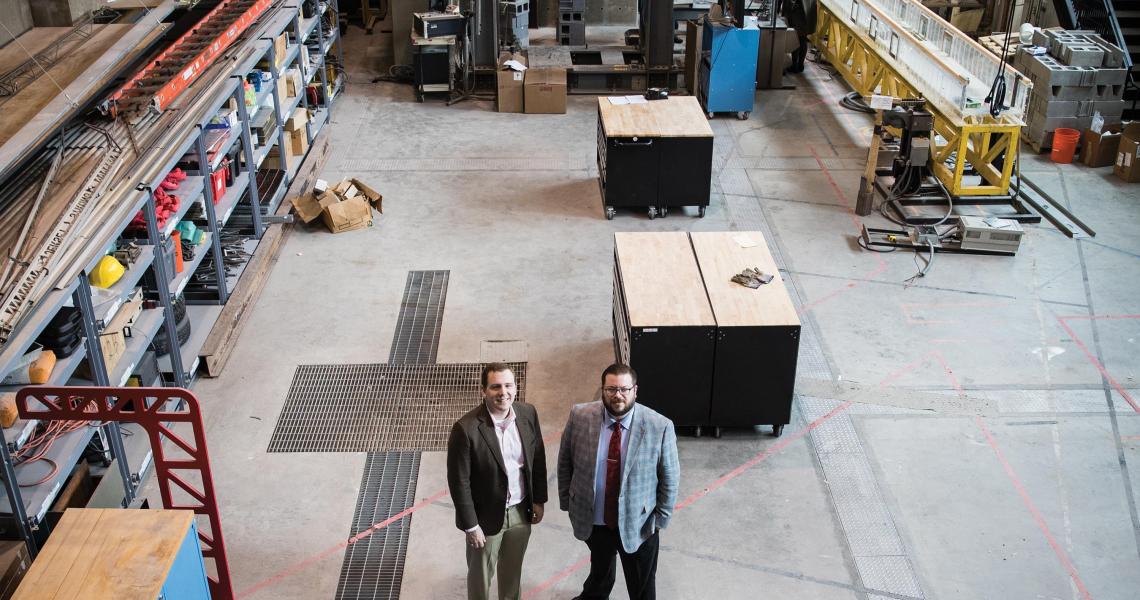
For an enterprising civil engineer in search of a challenge, there is no shortage of opportunities in the 21st century. The severe demand placed on the country’s infrastructure by booming urban populations, environmental conditions related to climate volatility and years of disinvestment, strains not only time and resources, but imagination and forecasting ability as well.
Indeed, rethinking the material building blocks of civilization – the asphalt, concrete and steel that compose roads, bridges and tunnels – now requires an added dimension: the ability to make durability projections not just for the standard 50 to 75 years of service life, but into a future in which climate change has made performance dynamic and unpredictable, notes Matthew Adams, assistant professor of civil engineering. Some larger projects, such as hydroelectric power dams or iconic bridges, are expected to last up to 150 years, despite growing uncertainty over what environmental conditions those years may bring.
Adams has joined forces with Matthew Bandelt, an assistant professor of civil engineering, to attack the problem on two fronts. They are assessing the soundness of massive backlogs of infrastructure projects expeditiously through experimental testing across multiple scales, in combination with robust computational models to predict the durability of new construction materials under scenarios that vary widely in temperature, weather volatility, chemical applications such as de-icing salts and loading conditions.
They are currently taking part in a Federal Highway Administration research program designed to produce tools to conduct large-scale assessments of bridge infrastructure performance by analyzing data from a representative sample of highway bridges nationwide. The aim is to improve durability by using predictive and forecasting models on deterioration and maintenance that will optimize the management of bridges.
Their collaborators and lead investigators on the research project, dubbed the Long-term Bridge Performance Program, are asset management specialists at the Center for Advanced Infrastructure and Transportation at Rutgers University, who have been collecting data on corrosion from bridges across the country using non-destructive testing instruments that examine reinforcement and concrete.
“The goal is to better statistically model how bridges will behave based on observed chemical and mechanical loading,” Bandelt says. “Most assessments done now are based on observation – it takes time to conduct scientific tests. We will evaluate their condition on a scale of one to ten and then examine mechanical and chemical factors. Taking their environmental loading into account, we will measure how bridges have actually weathered as compared to predictions.”
Adams will use this long-term performance data to determine how quickly various deterioration processes (corrosion, drying shrinkage, atmospheric carbon dioxide absorption, de-icing chemicals) and environmental loading (freeze-thaw cycles, temperature swings, proximity to salt water) affect the integrity of the bridges. Bandelt will use this information to create verified deterioration models for existing infrastructure that can then be applied to other systems across the country. “These models can also be linked to structural loading models to determine the impact of loads such as traffic on these systems as they deteriorate,” he notes.
Outside of the bridge project, Adams and Bandelt are examining fundamental questions about infrastructure performance.
“How do we create infrastructure capable of surviving a catastrophic event? How do we expeditiously replace it if it fails, and, more generally, how do we design and sustainably construct it with materials that are both cheaper and more durable?” Adams says. “Going forward, we can use computer models to see how stress builds up and materials deform as a result. This will let us design the appropriate materials for particular settings.
The novelty here is our collaboration,” he adds. “Historically, this work has been done in silos, but we’re trying to link across scales to characterize and quantify distinctions between the impacts caused by environmental conditions and mechanical loading, for example.”
Bandelt focuses on large-scale and component analysis, such as how building materials and structures respond to physical stress or “the damage a hurricane might cause.” He is creating computational models to determine how structural components will behave under a variety of loading scenarios, while also trying to predict where they will function best.
Adams looks at smaller-scale factors, such as the interactions over time of chemical systems in concrete and their impact on long-term durability and resiliency.
“I’ll put samples through 300 freezing and thawing cycles in a matter of weeks,” he says.
He looks at the use of new combinations of construction materials, including recycled concrete aggregates (RCA) functioning as the rocks in new concrete, and their impact on drying shrinkage, for example, which occurs when concrete loses water.
“Our results have shown that when you use RCA, systems can actually sustain higher levels of shrinkage prior to cracking. My hypothesis is that this is due to a deformation compatibility between the RCA and the new concrete. This means that we may actually be able to use RCA, a sustainable material, to create a more durable concrete. More generally, the new forms of fiber and recycled materials we put into concrete can affect both its internal chemistry and its physical structure and we need to know how it will perform,” Adams says. “Matt Bandelt has the ability to model some of these dynamics in ways that we can’t physically test, so that we can see how and when cracking occurs in different scenarios.”
In their newly refurbished laboratory, Adams and Bandelt can simulate up to 50 years of wear-and-tear under various environmental conditions such as freezing and thawing or exposing concrete to dry conditions. They have also acquired a new Forney compression machine that exerts up to 700,000 pounds of pressure to test strength as well as to look at the chemistry of a system.
Beyond simple strength testing, the compressor is able to “squeeze the juice,” as they describe pore solution, out of concrete samples. Using spectrometry, they can measure ionic activity, thus allowing them to track chemical changes in the system over time that result from their various conditioning methods.
“Making predictions when we don’t know what climate or loading conditions the infrastructure will experience is difficult,” Bandelt says. “However, because concrete is used all over the world, existing bridges, roads, tunnels and buildings comprise a very useful laboratory for letting us know how concrete will perform in all sorts of conditions. Combining this with information from climate- and trafficprediction models developed by other scientists, we can begin to develop high quality assumptions on what will happen to our new infra-structure.