Delving Deeper into the Circadian Rhythms of Life
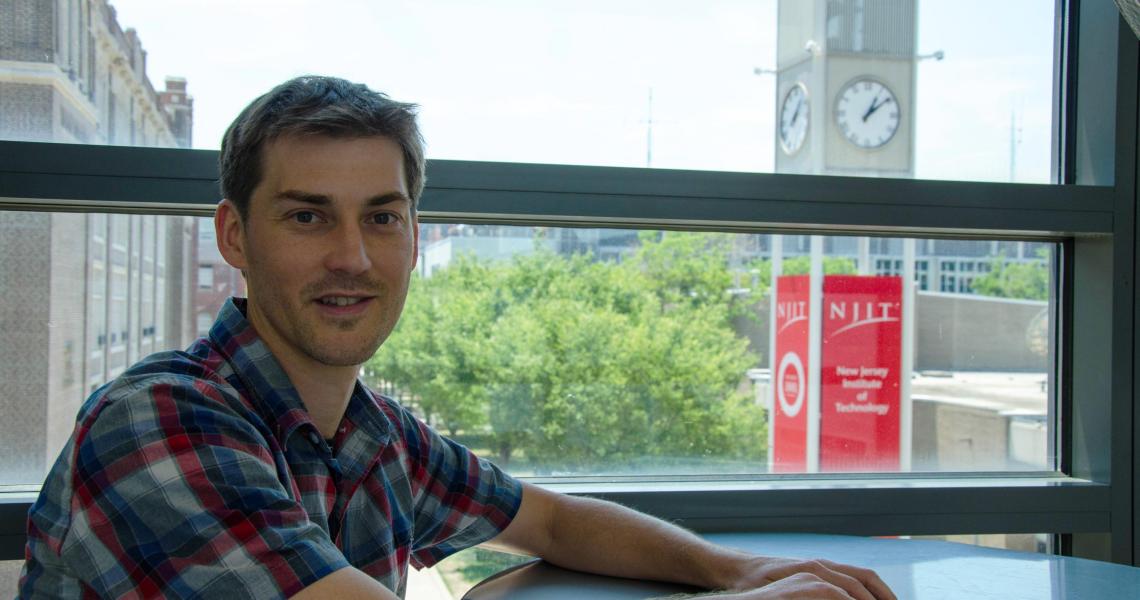
The circadian rhythms that harmonize our behavior with the daily cycle of light and dark, and with seasonal change, are among the most powerful physiological forces that we experience each day — forces that are experienced not only by other mammals, but also by many other living organisms.
The circadian behavioral imperatives programmed by evolution are also increasingly challenged by our culture. It’s why traveling quickly across multiple time zones causes jet lag, why shift work can affect our physical well-being, and why our mood and alertness in general can vary significantly in the course of the day. There may even be a circadian influence on why serious cardiac events are more likely to happen at certain times of the day, and why chemotherapy may be more effective if administered at certain times.
Although research has steadily added to knowledge about circadian behavior, our understanding is still far from comprehensive. Among the investigators working to advance what we know with new tools for modeling circadian processes at cellular and behavioral levels is Assistant Professor Casey Diekman, Department of Mathematical Sciences. Diekman has been at work in this area since joining the NJIT faculty in 2013, and he has received substantial funding for the effort, which could also contribute to the overall success of the U.S. BRAIN Initiative. BRAIN is an acronym for Brain Research through Advancing Innovative Neurotechnologies.
Diekman’s funding includes a three-year grant of more than $233,000 that he received from the National Science Foundation (NSF) and a recent five-year continuing NSF CAREER grant, with some $115,000 awarded to date. The NSF’s Faculty Early Career Development (CAREER) Program offers the foundation’s most prestigious awards in support of younger faculty who, in building their academic careers, have demonstrated outstanding potential as both educators and researchers.
Beginning at the Neuronal Level
Continuing his work of the past several years, Diekman is looking at neuronal activity in the suprachiasmatic nucleus, or SCN, which is a component of the brain consisting of some 20,000 neurons in the hypothalamus. The SCN receives information about the daily light-dark cycle from the external world through the retina, input that can affect circadian behavior. “The job of this part of the brain is to know what time of the day it is,” Diekman says. But the SCN can also perform this function without direct exposure to external light-dark conditions, and it is this capability that can be linked to conditions such as jet lag.
Diekman explains that this may very well be part of our evolutionary heritage, possibly associated with the evolution of distant mammalian ancestors that spent daylight hours inactive in dark spaces as a way to avoid predators, and then “instinctively” emerged at night to forage safely for food. “You want a system that is robust enough so that the internal sense of time will override confusing ‘noise’ in the environment, such as heavy cloud cover, yet at the same time respond appropriately to changes in the environment. So we experience jet lag because the robustness of the clock that serves us well under some circumstances when it comes to ‘noise’ also has ‘inertia’ which can cause us to lag in adjusting to environmental changes.”
Our circadian behavior over the course of the day is the cumulative product of the interplay of ionic currents within SCN neurons that generate electrical activity. This activity, which occurs on a millisecond time scale, is influenced by daily oscillations in gene expression within the SCN. Gene expression is the process by which DNA is translated into proteins, and proteins are the engines of most physiological functions, including circadian behavior.
Experimental neuronal data for Diekman’s mathematical modeling comes from Professor Hugh Piggins and Research Associate Mino Belle at the University of Manchester in England. A key objective for Diekman and Matthew Moye, a graduate student in Mathematical Sciences at NJIT, is to integrate this data into an increasingly comprehensive physiological model that simulates neuronal activity on the order of microseconds and clarifies the role of various ionic currents in daily patterns of circadian behavior.
Enlisting Drosophila
While Diekman is still working with data generated by the complexities of the circadian clock in mammals, he has broadened his experimental horizon by enlisting a new ally in the laboratory — Drosophila, more commonly known as the fruit fly. Over the decades, research involving fruit flies has yielded important insights into various aspects of biology, especially genetics. Now, in collaboration with Professor Ravi Allada and Research Associate Matthieu Flourakis at Northwestern University, studying the electrophysiology of circadian pacemaker neurons in Drosophila could advance our understanding of circadian behavior still further.
On one level, Diekman is continuing to develop models that mathematically detail the functioning of individual cells in the mammalian SCN. But constructing a more comprehensive picture of circadian phenomena requires insight into how the 20,000 cells of the SCN interact at the network level to influence behavior.
Diekman explains that the circadian clock in fruit flies shares many characteristics with the mammalian system. But there are just 150 neurons in a fruit fly’s circadian neuronal network. This relative simplicity promises to facilitate investigation of phenomena such as how neurotransmitter signaling molecules enable individual cells to synchronize with each other in response to changes over the light-dark cycle. This will also entail modeling the distinctive characteristics of seven different groups of cells, or clusters, in the fly’s circadian network and the functional connections among them.
“The goal is to build models of the complete fruit-fly clock, including detailed molecular and physiological models, and to test our models in the laboratory by measuring how long it takes the flies to respond to variations in the light-dark cycle,” Diekman says. “We believe that experimentally replicating what happens when we travel across time zones, for example, will help to answer significant questions about entrainment, how our physiology and behavior synchronize with environmental cues or at times are more in synch with what our internal clock tells us.”
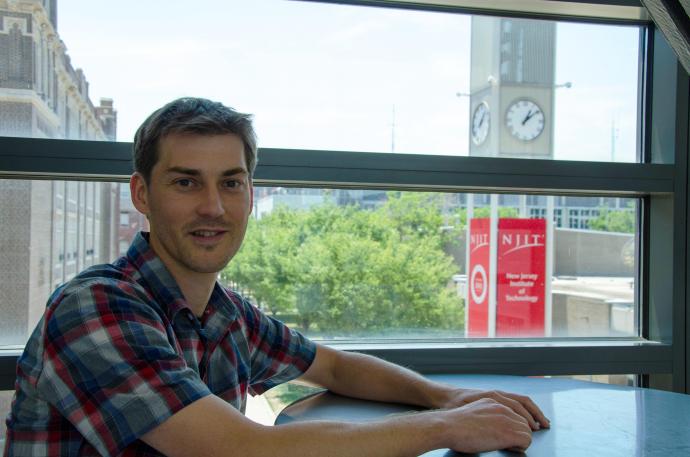
In their 2015 paper in the journal Cell, “A Conserved Bicycle Model for Circadian Clock Control of Membrane Excitability,” Diekman and researchers in Allada’s lab reported that increased sodium leak conductance depolarizes Drosophila circadian pacemaker neurons in the morning, leading to high electrical activity at that time of day. In the evening, increased potassium conductance hyperpolarizes these neurons and silences electrical activity. Remarkably, antiphase cycles in sodium and potassium conductances also drive membrane potential rhythms in mouse circadian clock neurons. Thus, this “bicycle” mechanism of controlling membrane excitability is an evolutionarily ancient strategy for governing daily sleep and wake behavior.
Broader Circadian Perspectives
When asked about future directions for circadian research, Diekman says that he and Professor Amitabha Bose, an NJIT Mathematical Sciences colleague, are developing a new mathematical tool for predicting the phase of our circadian entrainment called an “entrainment map.” Their approach allows them to map physiological responses to factors such as light intensity and seasonal changes in light duration directly to a mathematical function that determines the phase of entrainment.
On a daily basis, our phase of entrainment has significant implications reflective of both nature and culture. Most of us have to contend with jet lag only occasionally. A greater number of individuals experience the effects of shift work. But delayed circadian adjustment to “springing ahead” only one hour for daylight saving time may adversely affect many more people, and contribute to the statistical spikes in traffic accidents and workplace injuries that occur at this time of year.
Diekman says he is also intrigued by research indicating that circadian rhythms are integral to the physiology of cells throughout our body, not just in the SCN. These pervasive rhythms, involving variations in cellular activity, could explain why certain cancers seem to be more susceptible to chemotherapy at particular times of the day, and why sudden cardiac arrest appears to occur more frequently late in the morning and early in the evening.
Circadian rhythms are found not just throughout the animal kingdom, but also in plants and certain types of bacteria. Diekman, in collaboration with Professor Horacio Rotstein and graduate student Emel Khan from Mathematical Sciences, is developing mathematical models of the circadian clock in cyanobacteria. These models will be used to generate hypotheses about entrainment mechanisms that will be tested through experiments in the laboratory of Professor Yong-Ick Kim, NJIT Department of Chemistry and Environmental Science.
“I’m always looking for new applications of the tools that I develop and the models that I build,” Diekman says of his current research and potential future areas of investigation. It’s an effort likely to yield new understanding of the clock that links us to our evolutionary past and continues to time significant aspects of daily life.
By Dean Maskevich